With path splitter and recombiner constructed from time evolutions of interacting many-body system, nonlinear interferometry can achieve measurement precision beyond classical limit through preferential quantum amplification of signal over noise. Nonlinear interferometers are robust to detection noise, thus can overcome a major limitation to entanglement enhanced linear interferometry, that is highly anticipated to reach quantum advantage for the next generations of optical atomic clock, LIGO, and atomic magnetometer. The group of Prof. Li You from the department of physics, Tsinghua University, reported a novel approach to implement nonlinear interferometry with atomic spinor Bose-Einstein condensate (BEC). The work titled “Nonlinear interferometry beyond classical limit enabled by cyclic dynamics” was published in Nature Physics on December 20th.
Interferometer is a precise phase measurement apparatus. A typical interferometer consists of path splitter, phase interrogation, and path recombiner. Depending on their constructs, interferometers can be divided into two categories: linear and nonlinear ones. The well-known Michelson, Fabry-Perot, and Mach-Zehnder interferometers can be regarded as extensions of the Young’s double slit interferometer, that belongs to the category of linear interferometers due to their linear transformation properties of the associated path splitters and recombiners. As a result of the Heisenberg uncertainty principle, precision of a linear interferometer is lower bounded by classical limit, or standard quantum limit (
), when
independent particles are used. The major focus of quantum metrology is to overcome classical limit and to achieve higher precision with given resource. This could significantly boost the development of practical quantum technologies.
The traditional method for surpassing classical precision limit relies on the use of entangled particles as input. The nonclassical correlations among particles suppress quantum shot noises from measurements on individual particles, leading to improved signal-to-noise ratio. By driving through quantum phase transitions, You’s group deterministically prepared entangled atomic twin-Fock state witnessing over 910 plus particle entanglement in 2017 [Science 355, 620], and three-mode Dicke state with more than 10000 atoms fully entangled in 2018 [PNAS 115, 6381]. In 2021, they employed deep reinforcement learning to improve the entangled state preparation [PRL 126, 060401] and further improved world leading number squeezing of 13 dB (20 dB after subtracting known detection noise). Despite the tremendous success in the generation of entangled states, it is hard to unlock their high potential in metrological performance which requires low-noise detection technology.
Nonlinear interferometry can overcome the limitation of detection noise. Recent studies find when the operation of path recombiner is chosen as the time reversal of the nonlinear path splitter, the interferometry can realize preferential quantum amplification of signal over noise, also leading to enhanced signal-to-noise ratio. The amplification of quantum noise makes it robust to detection noise. However, it is generally difficult to realize time-reversed operation in a many-body interacting system, which constitutes a major obstacle in the development of nonlinear interferometry.
To solve this problem, You’s group devised a new approach for implementing nonlinear interferometry by making use of inherent cyclic dynamics of an interacting system, which is a ubiquitous feature when the many-body interacting system is integrable. Figure 1a shows vividly the difference between this method and the traditional one with sign flipped interaction. As shown in the left panel, for the traditional nonlinear interferometry based on time reversal, the initial classical state
evolves into an entangled probe state
after nonlinear path splitter
(denoted by solid blue arc). In the absence of phase interrogation, the subsequent path recombiner
(dashed blue arc) reverses dynamics and brings the system back to the initial state, while any minute phase perturbation in between the evolutions leads to a significantly deviated final state (yellow arc), which facilitates phase amplification. The right panel illustrates that inherent cyclic dynamics also brings the system back to the initial one, with any non-zero phase analogously causing the final state to deviate from the one without phase. This similarity implies the possibility of replacing time-reversed operation by the complementary time-forward nonlinear dynamics. You’s group demonstrates such a nonlinear interferometry in spin-1 87Rb atomic BEC, where competition between spin-exchange interaction and quadratic Zeeman energy gives rise to rich nonclassical physics. In 2005, Ph.D. student Wenxian Zhang (now a Professor at Wuhan University), postdoc Duanlu Zhou (now a scientist at Institute of Physics of the Chinese Academy of Sciences), and Prof. Li You proposed the mean-field inverted nonrigid pendulum model to describe the macroscopic periodic oscillation behavior.
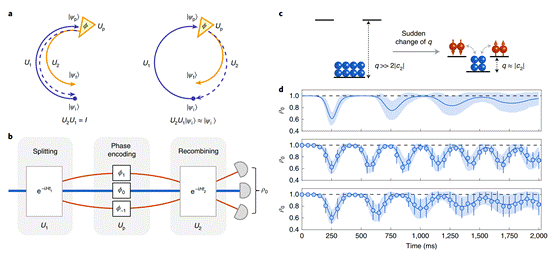
Figure 1. Nonlinear interferometer based on cyclic dynamics. a, Comparisons between the two types of nonlinear interferometry described. b, Illustration of three-mode nonlinear interferometer. c, Coherent spin oscillation induced by quenching quadratic Zeeman energy q to the vicinity of spin-exchange rate |
. d, Evolution of fractional population in
Zeeman spin component.
In the experiments, by precisely controlling atomic spin mixing dynamics with microwave and magnetic field, You’s group observed long-term coherent spin oscillation lasting over 2 seconds in a 87Rb atomic BEC consisting of 26500 atoms in the ground hyperfine state (Fig. 1d). To implement the interferometer, a phase to be measured is interrogated by pulsing on quadratic Zeeman energy with microwave field during the spin mixing dynamics (Fig. 2a). Based on the measurement of mean value and standard deviation of the fractional population in
Zeeman spin component, they achieved a metrological gain of 5 dB beyond the standard quantum limit (Fig. 2d-f). In this new protocol, the probe state for phase sensing is an entangled non-Gaussian state generated by long-term nonlinear evolution, which is strikingly different from squeezed states with Gaussian statistics generally utilized in linear interferometers. Therefore, this work also demonstrates the important role of nonlinear readout in the investigation of such non-Gaussian states for the first time.
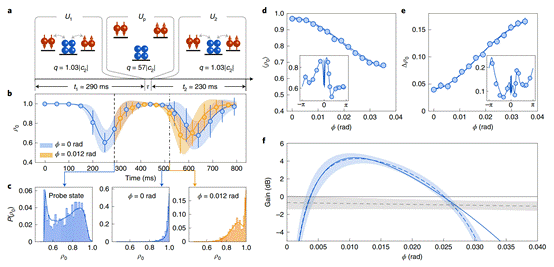
Figure 2. Phase sensitivity. a, Sequence of the nonlinear interferometer. b, Effect of phase interrogation on spin mixing dynamics. c, Probability distributions of the non-Gaussian probe state (left panel) and the final states after nonlinear readout (middle and right panels). d-e, Dependence of mean value and the standard deviation of
on interrogated phases. f, Phase sensitivity of nonlinear interferometer (blue lines) and three-mode linear interferometer (gray lines). Gains larger than 0 dB signify measurement precision beyond standard quantum limit.
This work is supported by the National Key R&D Program of China, by the National Natural Science Foundation of China (NSFC), by Tsinghua University, BAQIS, and Guangdong province.